Introduction
Organic Rankine Turbines (ORCs) are used to recover low temperature heat and produce electrical power. Organic fluids have a low freezing point, which make them very useful as working fluids in our cold Alberta climate.
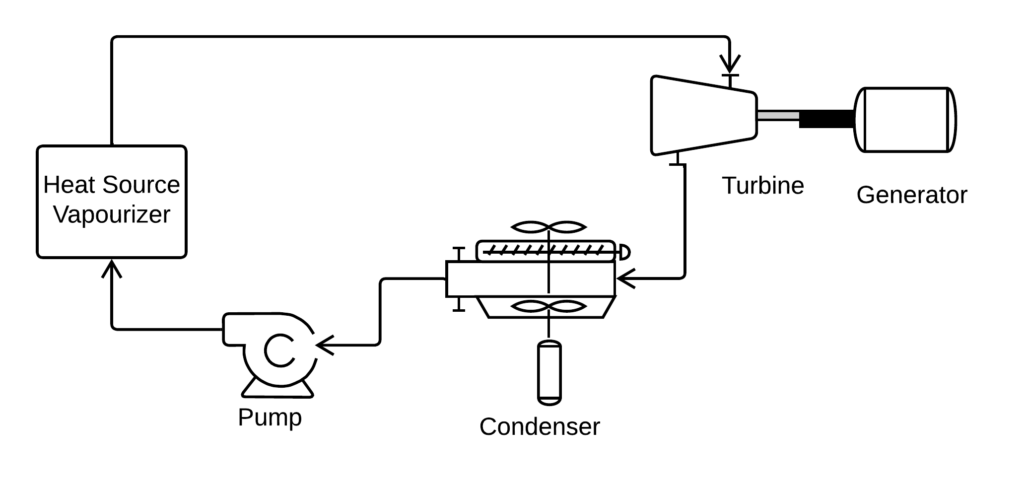
So-called Dry fluids such as nHexane are often used because they don’t form damaging mist at the turbine exhaust. However, we could also use a so-called Wet fluid, such as methanol, if we superheat the vapour.
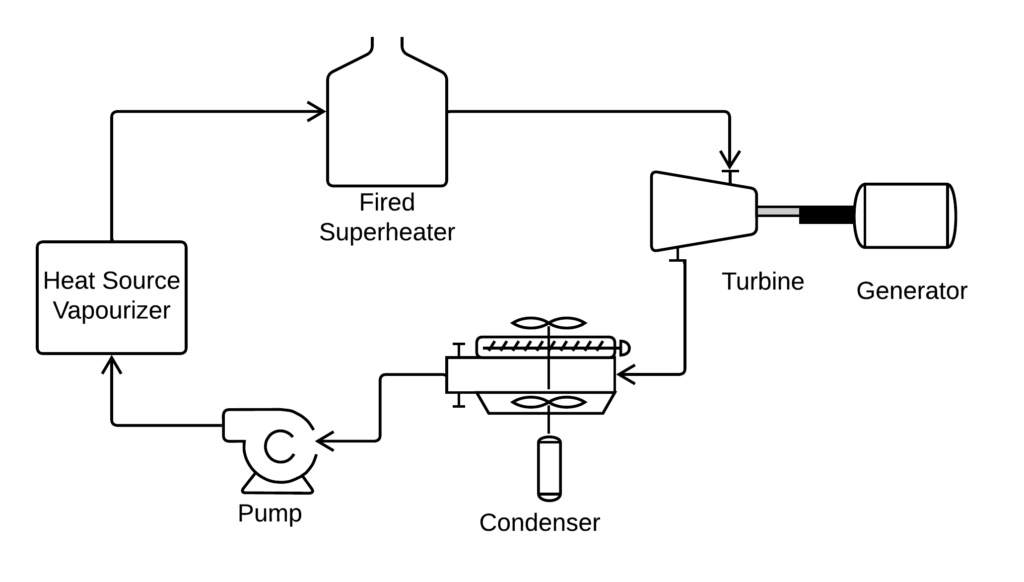
Would a gas fired superheater result in lower global CO2 emissions compared to an ORC that uses a Dry fluid? Could this be a valuable design for utilizing the low temperature geothermal resources in North west Alberta [ref 1]
A fired superheater has been demonstrated with Indian Point 1 nuclear power plant [ref 2]. In this plant, 2.5 MPA steam was heated with an oil-fired superheater from saturation 234 C to 540 C and delivered to a turbine.
This is a conceptual evaluation of an ORC with a fired superheater using methanol compared to a conventional ORC with a dry fluid n hexane. The results should indicate the relative merits of using a fired superheater with an ORC that uses geothermal heat.
Fluid properties
Physical properties of n-Hexane and Methanol are tabulated below
Fluid | Dry n-Hexane | Wet Methanol |
---|---|---|
Normal boiling temperature, C | 69 | 65 |
Critical temperature, C | 234 | 240 |
Critical pressure, kPaa | 3020 | 8100 |
Latent heat, kJ/kg at standard conditions | 360 | 1175 |
Thermodynamic diagrams (P-S) for nHexane and Methanol are shown below. Diagrams were created with CoolProp.
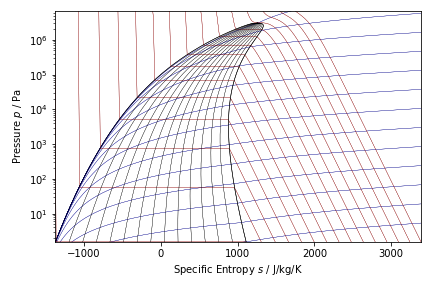
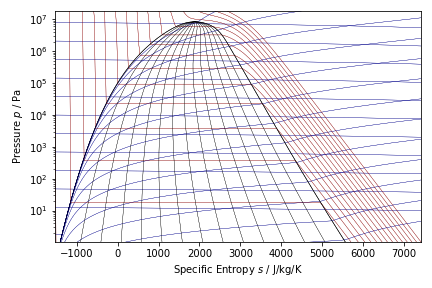
Results
Thermodynamic calculations are done with the Python version of CoolProp in a Jupyter Notebook [ref 3]. This is a great tool for exploring the thermodynamic properties of fluids and power cycles. CoolProp is open source and is comparable to NIST REFPROP [ref 4].
CoolProp has a feature for plotting a closed Rankine power cycle, but this failed for nHexane and methanol. The Rankine cycle for water is shown below.
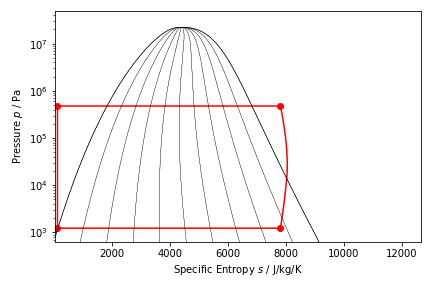
I will show the manual calculation steps. Recall that we need two unique process conditions to specify a thermodynamic state.
Dry ORC
- Fluid is nHexane
- Calculate the bubble pressure of the fluid at 150 C. This is the vaporizer pressure.
- Point 0. Condensed fluid. Quality is zero, temperature is 10 C.
- Point 1. Pump discharge. Pressure is the calculated vapouriEr pressuee, entropy is the same as S(0).
- Point 2. Vaporizer outlet. Pressure is the calculated appetizer pressure, quality is 1.
- Point 3. Turbine outlet. Pressure is P(0), entropy is S(2).
- Point 4. Vapourizer bubble point. Optional Calculation. Pressure is P(1), quality is 0.
- Point 5. Condenser dew point. Optional calculation. Pressure is P(0), quality is 1.
Results for dry ORC with n-Hexane.
Num | State | Q | T | P | H | S |
---|---|---|---|---|---|---|
0 | Condenser Bubble | 0.0 | 283.150000 | 10086.897029 | -137292.760223 | -439.403845 |
1 | Pump Discharge | -1.0 | 283.339065 | 753322.376106 | -136181.027642 | -439.403845 |
2 | Vapourizer Dew | 1.0 | 423.150000 | 753322.376106 | 478759.144684 | 1183.868594 |
3 | TD Exhaust | -1.0 | 336.313409 | 10086.897029 | 330128.352654 | 1183.868594 |
4 | Vapourizer Bubble | 0.0 | 423.150000 | 753322.376106 | 219218.652850 | 570.515193 |
5 | Condenser Dew | 1.0 | 283.150000 | 10086.897029 | 238893.579201 | 889.172314 |
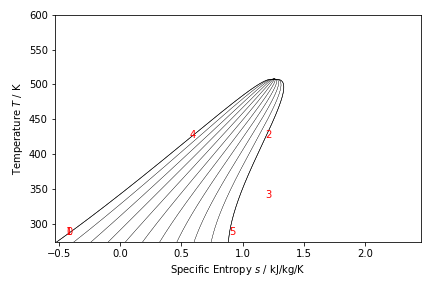
Superheated ORC
- Fluid is Methanol
- Calculate the bubble pressure of the fluid at 150 C. This is the vaporizer pressure.
- Point 0. Condensed fluid. Quality is zero, temperature is 10 C.
- Point 1. Pump discharge. Pressure is the calculated vapouriEr pressuee, entropy is the same as S(0).
- Point 2. Vaporizer outlet. Pressure is the calculated vapourizer pressure, quality is 1.
- Now we change the calculation sequence.
- Point 4. Turbine outlet. Pressure is P(0), quality is 0.9.
- Point 3. Superheater Outlet/Turbine Inlet. Pressure is P(2), entropy is S(4).
- Point 5. Vapourizer bubble point. Optional Calculation. Pressure is P(1), quality is 0.
Num | State | Q | T | P | H | S |
---|---|---|---|---|---|---|
0 | Condenser Bubble | 0.0 | 283.150000 | 7.400186e+03 | -1.429067e+05 | -460.195881 |
1 | Pump Discharge | -1.0 | 283.383855 | 1.393475e+06 | -1.411766e+05 | -460.195881 |
2 | Vapourizer Dew | 1.0 | 423.150000 | 1.393475e+06 | 1.127459e+06 | 2732.638884 |
3 | SH Vapour | -1.0 | 516.718172 | 1.393475e+06 | 1.404935e+06 | 3335.987036 |
4 | TD Exhaust | 0.9 | 283.150000 | 7.400186e+03 | 9.319825e+05 | 3335.987036 |
5 | Vapourizer Bubble | 0.0 | 423.150000 | 1.393475e+06 | 2.786294e+05 | 726.660532 |
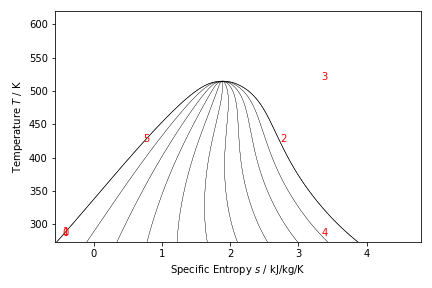
Result Comparison
Process | Dry n-Hexane | Superheated Methanol |
---|---|---|
Geothermal Heat, MW | 10 | 10 |
Mass Flow, kg/s | 16.3 | 7.9 |
Vapourizer temperature, C | 150 | 150 |
Vapourizer pressure, kPa | 753 | 1393 |
Superheat duty, MW | N/A | 2.2 |
Superheat temperature, C | N/A | 244 |
Turbine Power, MW | 2.4 | 3.7 |
Turbine Exhaust Temperature | 63 C (53K superheat) | 10 C (90% quality) |
Condenser Temperature, C | 10 | 10 |
Condenser Pressure, kPa | 10 | 7.4 |
Condenser Duty, MW | 7.6 | 8.5 |
Analysis
The ORC with nHexane produces hot exhaust from the turbine. This represents a loss of power produced by the turbine.
The superheated ORC consumes 2.2 MW of energy from fuel and produces an additional 54% (1.3 MW) of electrical power , compared to the dry ORC case. This means that 60% of the fuel heating value was converted to power, which is substantially better than 35% efficiency for a SCGT or 50% efficiency for a CCGT.
A fired superheater is inexpensive compared to the geothermal wells and turbine. Since the superheated turbineis 50% larger than the dry case, the superheated turbine is roughly 50% more expensive.
The relative capital costs for the dry and Superheated ORCs are
Process | Dry | Superheated |
---|---|---|
Geothermal well | 40 | 40 |
Turbine | 40 | 60 |
Everything else | 20 | 20 |
Total | 100 | 120 |
The superheated ORC facility should cost about 20% more than the Dry ORC facility.
We can estimate the amount of CO2 that is displaced by each of these facilities. For most of the year, the Alberta power grid relies on inefficient SCGT and gas-fired boilers to ensure that power supply meets demand. Thus, we will assume that the power generated by the ORCs will displace power generated by these inefficient sources.
The higher heating value of methane is 55,500 kJ/kg. This equates to 49.55 kg CO2 per GJ, or 178 kg CO2 per MW.hr.
Data from the EIA fuel heating rate (2022 data) [ref 5] shows that a simple cycle gas turbine has a specific heating rate of 11,030 HHV BTU per kW.hr. The is equivalent to 518 kg CO2 per MW.hr.
We compare the mass of CO2 that is displaced by the 2.4 MW dry ORC, with the 3.7 MW produced by superheated ORC and allow for the added CO2 produced by firing the superheater at 2.2 MW.
The superheated ORC reduces net CO2 emissions by 23% more than the dry ORC, even though we fired the superheater with natural gas. The analogy is the geothermal heat provides the heavy lifting, where the gas fired superheater adds value that becomes additional electrical power.
Summary
Both of the ORCs are good for providing a base supply of low CO2 electrical power. The addition of this base supply reduces the dependancy on the existing gas fired SCGT and CCGT power plants. This means that the Alberta power grid can implement more Opportunistic power sources from Wind and Solar facilities and still have a reliable power grid.
The gas fired superheater results in greater reduction of CO2 emissions than the plain dry ORC. This is due to the high efficiency of converting energy from superheated gas to shaft power.
A superheated ORC could be a good fit for Alberta in the quest for low cost power plants that utilize renewable energy and provide a reliable supply of low cost power.
References
- Alberta geothermal temperature map. https://ags.aer.ca/research-initiatives/geothermal-temperature-mapping
- NOAM LIOR, ENERGY, EXERGY AND THERMOECONOMIC ANALYSIS OF THE EFFECTS OF FOSSIL-FUEL SUPERHEATING IN NUCLEAR POWER PLANTS, Energy Convers. Mgmt Vol. 38, No. 15-17, pp. 1585-1593, 1997
- CoolProp,http://www.coolprop.org/
- Bell, Ian H. and Wronski, Jorrit and Quoilin, Sylvain and Lemort, Vincent
- Pure and Pseudo-pure Fluid Thermophysical Property Evaluation and the Open-Source Thermophysical Property Library CoolProp
- Industrial & Engineering Chemistry Research, 53 (6), pp 2498 – 2508, (2014)
- NIST REFPROP,https://www.nist.gov/srd/refprop
- EIA data, 2022. https://www.eia.gov/electricity/annual/html/epa_08_02.html